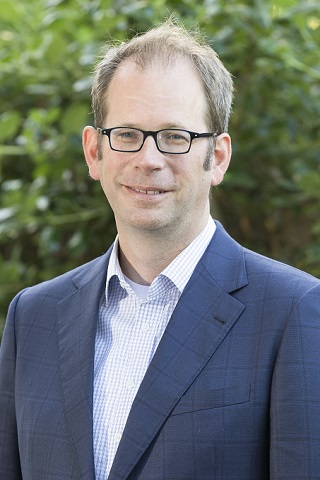
Eric Gimon
Green hydrogen has catapulted into the limelight thanks to grant funding and tax credits in the recent U.S. Inflation Reduction Act (IRA) and newly announced mega projects like the multi-billion-dollar AES/Air Products facility in Texas. This surge in interest isn’t just a trend; it reflects a deeper shift in our energy landscape. As clean electricity increasingly becomes the backbone of our energy grid, green hydrogen also aims to support decarbonization of other areas of the economy like the production of fuels and chemical feedstocks.
In the context of a low-carbon economy, hydrogen – particularly “green” hydrogen produced from clean electricity – emerges as a critical intermediary linking the electricity grid to new sectors.
However, just because something can be done, doesn’t mean it should be done. Obviously, making “dirty” hydrogen from greenhouse gas–emitting fossil fuels runs counter to the goal of decarbonization, but even converting clean electricity into other forms of energy (and back) can waste significant energy. While fuels traditionally lose energy when converted to electricity (40-70% efficient), the tables are turned with fuels or feedstocks produced from electricity. For applications where clean electricity can only functionally compete with mainstream chemical feedstocks and fossil fuels after being converted into an intermediate product such as hydrogen, it is now the source facing an efficiency penalty from conversion. So for clean electricity derived products to achieve cost parity with incumbent fuels, further cost reductions in conversion equipment like electrolyzers and ready access to the cheapest source of clean electricity are required.
Amidst the growing hype around hydrogen, it’s crucial to acknowledge these efficiency challenges. Hydrogen is not a one-size-fits-all solution. Still, despite efficiency limitations, there remains a large potential market for clean hydrogen in a decarbonizing world, substantially larger than today’s dirty hydrogen supply, for specific applications when other alternatives for fossil fuels and chemical feedstocks aren’t available.
This blog post delves into the key aspects of efficiency, cost, and the enormous energy consumption required to transform electricity into material amounts of hydrogen. Much like how scientists begin massive projects like atom-smashers or space telescopes with simple back-of-the-envelope calculations to gauge feasibility, we’ll apply the same approach to explore the potential and limitations of green hydrogen in the context of the electricity grid.
Hydrogen as Long Duration Storage
One common example of clean fuels interacting with the grid is green hydrogen for long-duration storage. The process involves converting electricity and water to hydrogen via electrolysis and then back to electricity using combustion or a fuel cell (see Fig. 1). The initial step, splitting water into oxygen and hydrogen gas, uses about 40 kWh of electricity to produce one kg of hydrogen (high heating value). The best commercial electrolyzers operate at around 50 kWh/kg H2, achieving an efficiency of just under 79%, with more improvements possible.
After some time in storage, hydrogen combusts or runs through a fuel cell, typically generating steam as a byproduct. Steam as an output incurs a nearly unavoidable 15% efficiency loss (useful electricity output maxes out at the low heating value). Combustion turbines offer 42-44% efficiency in single-cycle mode and up to 64% in combined-cycle mode, albeit not with 100% hydrogen. Fuel cells vary from 50-70% efficiency.
Combining these factors yields a round-trip efficiency for hydrogen as long-duration storage as shown in the chart above. For every MWh of electricity returned during peak times from hydrogen as long-term storage, 2 to 3 MWh are lost. From a market operation perspective, this means that energy bought at $20/MWh needs to be resold at more than $60-80/MWh to be economically viable. This dynamic means that hydrogen will not compete well with lithium-ion batteries, which have 80-90% efficiency. However, for about 5% of annual peak hours, hydrogen could be economically feasible when purchasing energy for less than $20/MWh.
This economic analysis doesn’t factor in the capital costs of electrolyzers and turbines. This omission is intentional, to highlight the underlying marginal cost mathematics independent of capital expenditure. While there will be equipment cost reductions and near-term help from the IRA’s lucrative federal subsidies, round-trip efficiency will remain key to the fate of hydrogen-as-storage.
If hydrogen-based long-duration storage achieves 45% round-trip efficiency, it will be comparable to competitors in long-duration storage like Form Energy’s iron-air battery. Additionally, hydrogen offers the advantage of being a fungible good. Unlike other electricity storage systems where the quantities of storage medium produced are directly linked to the anticipated electricity use, hydrogen can be traded on the open market. This flexibility means that an electrolyzer/peaker combo can produce excess hydrogen at the electrolyzer when input power is cheap (selling off surplus hydrogen) or buy additional hydrogen peaker fuel when grid conditions are tight.
Hydrogen for Fuel or Chemical Feedstocks
Moving beyond electricity storage, hydrogen electrolysis economics can dictate limits on hydrogen’s role in the fuels and materials worlds.
Green hydrogen could compete with the existing U.S. and international markets for hydrogen as a chemical feedstock. Extending to fuels sectors, it’s important to note that buying hydrogen at $1/kg means buying energy at $8 per million Btus (MMBtu). To compete with natural gas at current U.S. market prices, hydrogen needs to be priced around $0.35/kg. For other fuels the price target can be less daunting. Accounting for further production costs, converting $1/kg hydrogen to e-kerosene could compete with traditional sources. Similar calculations apply in conversations about hydrogen in other industrial processes or heat. The U.S. Department of Energy’s price target for unsubsidized clean hydrogen is $1/kg by 2031, competitive with today’s “grey” hydrogen produced through steam methane reformation.
Apart from costs, in thinking about green hydrogen production it is important to realize that substantial quantities of hydrogen – millions of metric tons (MMT) per year – would be required to participate materially in existing fuel and feedstock markets. For example, current U.S. consumption of kerosene, the main source of jet fuel, is around 25 billion gallons per year. Hydrogen sufficient to replace all 25 billion gallons with sustainable aviation fuel (SAF) requires 25 MMT, with increased electricity demand equivalent to 30% of all the electricity generated per year in the U.S. today.
Sustainable feedstocks made from hydrogen also involve immense amounts of electricity. For example, the U.S. produces about 13 MMT annually of ammonia, a staple in fertilizer production. Simple stochiometric bounds imply that the equivalent amount of green ammonia via hydrogen would require a minimum of 2.3 MMT of hydrogen. Replacing the U.S. annual blast-furnace steel production would involve about 1.5 MMT of hydrogen. Put all these pieces together, and there may be more demand for (green) hydrogen in a zero-carbon economy than the grid can provide without significant and sustained growth in clean generation over decades.
From a distribution perspective, hydrogen poses further unique challenges. A U.S. Department of Energy chart from the hydrogen lift-off report, Pathways to Commercial Liftoff: Clean Hydrogen, shows some of the storage and distribution options for hydrogen with cost for technology “at scale” (see summary in Fig 2). The cheapest combination of compression, storage, and transport costs still represents at least a $0.30/kg adder to the production price of hydrogen. For context, according to the Energy Information Agency, the U.S. average delivered natural gas for industrial customers was around $4.4 per Million Btu in November 2023, which beats hydrogen on price as an energy source anywhere above $.50/kg. This competition only leaves $.20/kg to cover the production costs of a commodity typically above $1/kg.
Policy Implications
- Long-Duration Storage: For hydrogen to be effective as long-duration storage, it requires a steady supply of low-cost input power, significantly lower than the prices at which it will sell peak power. This dynamic creates a wide price gap between electricity buying and selling, with a ratio of at least three to four times more when considering capital costs for electrolyzers, hydrogen storage, and hydrogen peakers or fuel cells. While it may make sense to direct support for hydrogen as a reserve or peaking resource, policies and resource plans aiming for more than a double-digit capacity factor from hydrogen-based generation should be avoided.
- Alternative Fuel or Feedstock: For hydrogen to compete in these sectors, the input power cost needs to be even lower, likely in the range of $10-20/MWh. To harvest such cheap power after tax credits expire, electrolyzers will need to operate flexibly at less than 20-30%. Policy efforts should focus on creating sustainable business models that accommodate these operational flexibilities.
- Economic Viability: Unless there’s a drastic reduction in electrolyzer prices, making it economically feasible for them to operate for a very limited fraction of time, hydrogen will struggle to compete economically with low-cost fossil energy sources in most applications, like building heat. In those contexts, direct electrification is usually a much cheaper way of achieving zero-emissions goals with the least consumer pain, as it can compete head-to-head with fossil fuels, and deserves a lot more policy-maker support. Carbon pricing or mandates could shift the balance, but just the costs of hydrogen transportation and storage on top of production costs are likely to mean that green hydrogen remains prohibitively expensive for many business cases. It should be seen more as a solution of last resort when direct electrification is not possible (e.g. long-haul aviation).
Conclusion
Exploring the impacts of hydrogen production on the grid reveals a landscape filled with potential and pitfalls. While green hydrogen offers new avenues for long-duration storage and an entry into the sustainable fuels and feedstocks market, its economic and environmental viability hinges on a multitude of factors. These include efficiency improvements, cost reductions, policy support, and innovative business models – but most of all requires a clear-eyed view of the key numbers involved. As the world moves towards a cleaner electricity grid, hydrogen stands as a promising yet challenging player, one that requires careful consideration and strategic implementation.
Eric Gimon
Senior Fellow
Energy Innovation LLC
Nice Information! Green hydrogen is feasible with declining renewable energy costs. Assuming an electrolyzer efficiency of 70%, and renewable energy costs at $0.03 per kWh, green hydrogen production could cost around $1.43 per kg, competitive with gray hydrogen.